By Kristine M. Larson, Eric E. Small, and John J Braun - Fall 2010
The EarthScope Plate Boundary Observatory (PBO) was created to study the motion of tectonic plates and the deformation of the North American continent. The GPS data collected as part of PBO, though, contain additional information that is very useful in ways unforeseen when the original PBO science plan was created. This article summarizes new ways of using GPS data to study soil moisture, snow cover, and biomass conditions.
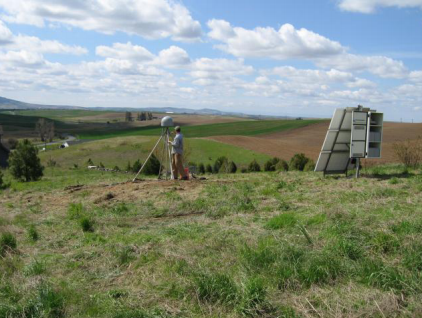
The PBO operates ~1100 continuously operating GPS stations and reaches its original goal of studying plate motions and continental deformation by measuring millimeter changes in the position of the GPS stations over time periods that range from months to years. In order to accommodate the needs of the EarthScope science community, UNAVCO developed an analysis plan that provides two kinds of GPS products. The first is a daily position value for the antenna – i.e. latitude, longitude, and height for each GPS site. The second product consists of velocity estimates for each site based on the daily positions, where we assume ground motion can be modeled linearly. In addition to the position and velocity products, geodesists also have access to and analyze the raw GPS observables and the measured distances between the GPS satellites and receivers.
Many factors influence raw GPS observables besides antenna position: satellite orbits, relativity, satellite and receiver clocks, tides, the atmosphere, and reflected signals. The last of these – also known as multipath – is the result of a signal that bounces before it arrives at the antenna. One can think of these reflections as weaker “echoes” of the desired direct signal. PBO does not try to remove the effects of multipath reflections, instead relying on instrument manufacturers who have designed both the GPS receiver and antenna to suppress them. Nevertheless, it is well known by geodesists that the effects of reflected GPS signals can still be observed in PBO data. Engineers actually design radars to detect reflected signals to monitor surface environmental conditions like soil moisture levels. The reflected GPS signal can be used in the same way – to use PBO as an environmental sensing network. Compared to other networks and satellites currently measuring such changes, PBO has the advantage of providing a homogeneous network over most of the western US combined with existing telemetry and archiving.
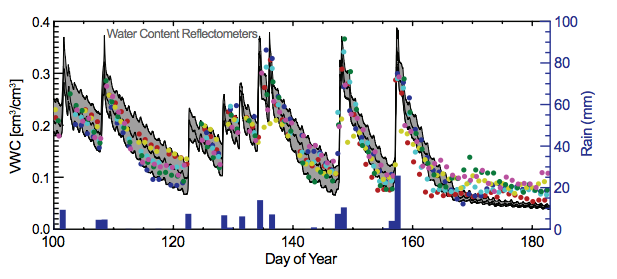
Radars take advantage of the fact that the radar signal penetrates and is reflected by dry soil differently than by wet soil, yielding estimates of shallow soil moisture. This principle is also true for GPS reflections. A radar on a satellite – such as the upcoming NASA SMAP mission (http:// smap.jpl.nasa.gov) – typically has a very large spatial footprint (25-600 km2). In contrast, in situ soil moisture sensors have a very limited spatial sensitivity (on the order of 100 cm2). The PBO GPS sites have a footprint of ~1000 m2, providing a bridge between in situ probes and satellites. Figure 1 shows the first demonstration that reflected GPS measurements correlate well with in situ soil moisture probes. The soil responds immediately to large precipitation events, followed by long dry-downs of days to weeks. Soil moisture data are important to weather forecasters, hydrologists, and climate scientists so that they can properly initialize their models.
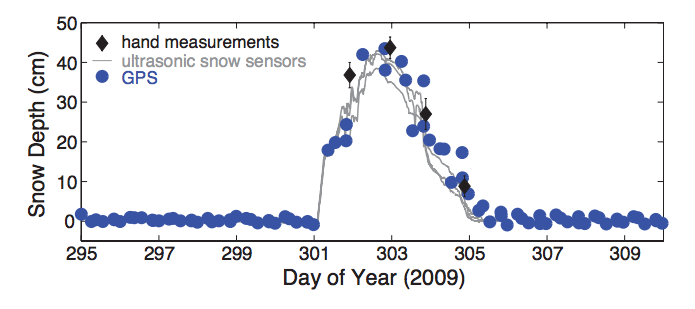
If GPS can detect when soil is wet vs. dry, it is not too surprising that we have also been able to detect snow. In fact, snow effects are far easier to measure with GPS reflections than soil moisture. Figure 2 shows GPS estimates of snow depth from a winter storm at a PBO site near Boulder, Colorado. The GPS method is able to capture both the rapid snow accumulation and the melting of the snow over two days. Although snow is currently measured by NOAA and state agencies, many of these measurements are either infrequent (monthly) or focus on very small footprints (10 m2). Using PBO to measure snow has the advantages of a large footprint, which provides a more representative measurement of snow accumulation, and of a continuously operating sensor. Snowpack and melt rate information are essential for management of the water supply and flood control systems, particularly in the more arid western US.
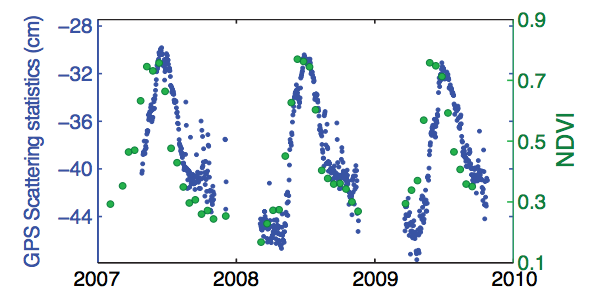
Most recently, our group showed that vegetation changes are also sensed by reflected GPS signals. In contrast to soil moisture and snow signals, which are dominated by coherent reflections, vegetation primarily scatters radar returns. Figure 3 shows daily multipath scattering statistics at a PBO site in western Idaho. There is a strong negative correlation between the GPS scattering statistics and vegetation indices as measured by satellite – consistent with expectations that when vegetation is high, fewer signals will be reflected back to the antenna. Estimates of vegetation state are required for hydro-meteorological modeling and for validation of satellite surveys of land surface conditions. We are currently conducting experiments at agricultural sites in Boulder County to further validate our results. For example, we will compare the height and water content of an alfalfa field, measured weekly by a field crew, with GPS scattering statistics.
The original science goals of PBO were very much oriented towards understanding deformation of the solid Earth. Recently EarthScope updated its science goals (www.earthscope.org/ESSP) to include water cycle studies. Observing and monitoring spatial and temporal changes in the water cycle are needed to understand and predict a future climate. This new EarthScope initiative is another demonstration of how PBO contributes to solving problems of societal importance and with significant consequences for our future. Our work also shows how development of infrastructure for one purpose can yield inadvertent and “free” science products for other disciplines. In fact, our studies using PBO data could be applied to other GPS networks globally, adding crucial water cycle and vegetation information at intermediate scales (1000 m2) augmenting existing in-situ and remote-sensing systems.
References
Larson, K. M., E. E. Small, E. D. Gutmann, A. L. Bilich, P. Axelrad, and J. J. Braun, Using GPS multipath to measure soil moisture fluctuations: initial results, GPS Solut., 12:173-177, doi: 10.1007/s10291-007-0076-6, 2008.
Larson, K. M., E. E. Small, E. D. Gutmann, A. L. Bilich, J. J. Braun, and V. U. Zavorotny, Use of GPS receivers as a soil moisture network for water cycle studies, Geophys. Res. Lett., 35, L24405, doi: 10.1029/2008GL036013, 2008.
Larson, K. M., E. D. Gutmann, V. U. Zavorotny, J. J. Braun, M. W. Williams, and F. G. Nievinski, Can we measure snow depth with GPS receivers?, Geophys. Res. Lett. 36, L17502, doi: 10.1029/2009GL039430, 2009.
Larson, K. M., J. J. Braun, E. E. Small, V. U. Zavorotny, E. D. Gutmann, and A. L. Bilich - GPS multipath and its relation to near-surface soil moisture content, IEEE J-STARS, 3, 91-99, doi: 10.1109/JSTARS.2009.2033612, 2010.
Small, E. E., K. M. Larson, and J. J. Braun, Sensing vegetation growth with reflected GPS signals, Geophys. Res. Lett., 37, L12401, doi: 10.1029/2010GL042951, 2010.
Zavorotny, V. U., K. M. Larson, J. J. Braun, E. E. Small, E. D. Gutmann, and A. L. Bilich - A physical model of GPS multipath caused by land reflections: toward bare soil moisture retrievals, IEEE J-STARS, 3(1), 100-110, doi: 10.1109/JSTARS.2009.2033608, 2010. doi:10.1029/2009GC002787, 2010.
Support
We have developed this research using funding from NSF AGS 0740515, AGS0740498, AGS 0935725, AGS 0935728, EAR 0948957 and two University of Colorado Interdisciplinary Seed Grants. Bob Munson of Munson Farms, Boulder, Colorado and Boulder County Open Space have graciously allowed us to install GPS receivers at their agricultural fields. We acknowledge archiving support from UNAVCO and especially thank UNAVCO’s Jim Normandeau and Sarah Doelger for field assistance. Penina Axelrad, Andria Bilich, Ethan Gutmann, Felipe Nievinski, Mark Williams, and Valery Zavorotny have provided valuable feedback.